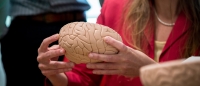
Guidelines for Brain Imaging of Children at Risk for Autism Spectrum Disorder
Kristina Denisova, PhD, an Assistant Professor of Clinical Neurobiology (in Psychiatry) at Columbia University Irving Medical Center, specializes in state of the art brain imaging of children at risk for Autism Spectrum Disorders (ASD). Studies in her lab are focused on producing new knowledge about how the mind develops in children in the hopes that her team’s efforts will accelerate discovery of the origins of atypical development and contribute toward the development of supports and individual interventions for these children.
The focal point of Dr. Denisova’s work is uncovering the underlying principles used by the brain for perception, action, and for the efficient interaction with the environment. She says one important and underappreciated secret of how our cognitive processes unfold is that not all signals that are received by our sensory organs, such as the eye or the ear, are processed by the brain. In fact, only a tiny portion of the total number of all signals are processed; the rest are discarded. Some signals are given priority in processing, guided by constraints on the signal representation, such as evolutionarily driven assumptions about our physical world. This is true for all sensory modalities. “How this representation occurs in the developing mind, and what are the variables that contribute to our cognition is a scientific puzzle that we are working on, with the hope to unravel how atypical brain development emerges in some children,” says Dr. Denisova. The Denisova lab currently pursues several research directions in this area, including continued work with typically developing children, using several analytic techniques including computational modeling and advanced neuroimaging. “We are now at the starting line for doing scientifically valid, neurobiologically-grounded, and conceptually advanced work in atypical development in humans,” Dr. Denisova said.
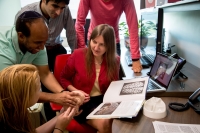
In-vivo, non-invasive magnetic resonance imaging (MRI) scans provide a distinct way to study some aspects of brain development and maturation, but care is warranted on how researchers use this technique in a still-developing nervous system. For example, atypically developing children move their heads more while being scanned in these fMRI studies, an issue that affects data quality and inference.
Dr. Denisova discovered the head movement problem while scanning children with ASD during her NIMH T32 fellowship at Columbia. Patients with ASD moved their heads more relative to typically developing children while being scanned, forcing her to exclude many participants’ data from final analyses. “As I became interested in studying whether infants solely at risk for autism also exhibit atypical head movements during the scans, I studied patterns of head movements from infants at high vs. low risk for ASD, and detected between-group differences as early as 1-2 months after birth,” she says.
Further, Dr. Denisova’s structural neuroimaging studies in children with ASD revealed important insights about potential substrates associated with ASD that are rooted in the cerebellum. Such experiences led Dr. Denisova to re-think her team’s approaches to how brain imaging is done in the very young who are at risk for atypical development and led, in part, to her invitation from the Guest Editors of the Special Issue in the NeuroImage: Imaging Baby Brain to help identify existing challenges and to formulate a set of state-of-the-art guidelines at the leading edge of our current knowledge in infant and children brain imaging. These guidelines are congruent with what is known about the developing mind to date and are centered around three key issues, with specific examples and solutions given for each of the issues in the article.
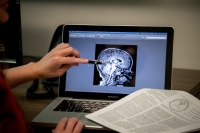
When imaging children with ASD, as well as those at risk for autism or atypical development, Dr. Denisova says there are three specific challenges for researchers: head movements, hemodynamics and tissue structure:
Head movements. Movements of the head during a neuroimaging scan present a problem when conducting studies with children. First, head movements distort the consistency of the magnetic field and affect the quality of the acquired data. To mitigate their impact, imagers apply stringent motion-correction strategies to ensure that mostly low-motion timeseries are used as input into statistical analyses. However, when working with more than one cohort (such as a low risk and a high risk infant group) and removing volumes in the timeseries that show excessive movement, caution is warranted as subtle differences in the contiguity of data segments from the original timeseries can exist between the two cohorts. Ignoring the possibility that different cohorts may contribute differently-sized segments to the timeseries used for the final statistical analyses may lead to what Dr. Denisova has termed as a BOLD mosaic effect (BOLD refers to blood oxygenation-level dependent T2* signal acquired using an EPI pulse sequence). Overall, this fragmentation effect may potentially lead to spurious seed-based brain connectivity findings in children and related cohorts. Thus, researchers are advised to use contiguous segments of data of similar length when analyzing fMRI or rs-fMRI data in studies involving several cohorts. Dr. Denisova also suggests longer-duration, flexible scan sessions for young children (instead of several, but shorter, runs of traditional length of 5 to 6 minutes).
Second, while problematic for neuroimaging analyses, head movements cannot be considered a trait in that some infants move “more” or “less”, as typically developing infants show dissociable head movement signatures to different stimuli. Dr. Denisova says that from a developmental perspective, “we know that a newborn has different sensorimotor behaviors while they are sleeping compared to when they are listening to language.” Yet, as work in her lab has found, this is not a correct assumption to make for infants at high risk for autism, as these infants have atypically similar head movements to diverse inputs. The important question is, why? This question is now propelling a transformation in the understanding of the roots of atypical development in young children, including a renewed focus on the importance of sleep for the developing brain. Further, one way to probe why some children show similar head movements may be to use quantitative MRI acquisitions (discussed below) while monitoring data quality during scan acquisition.
Hemodynamics. Functional MRI (fMRI) studies infer brain functioning by estimating or modeling the hemodynamic response to stimuli, but in early life, this response is not well-developed. It may be inappropriate to use a standard, adult Hemodynamic Response Function (HRF) when modeling brain function in infants and children, in particular when aiming to probe functional integrity of the deeper subcortical structures, which differ in neurovascular coupling and maturational trajectories relative to the cortical tissues. Instead, researchers may consider using a more individualized, infant- or child-specific shape of the HRF. Dr. Denisova says she hesitates to recommend studies in children involving resting-state fMRI (rs-fMRI) acquisitions, as we know virtually nothing about which bandwidth of signals we need to use when modeling these data in children. The issue is complex because rs-fMRI studies do not explicitly model the HRF. For example, the widely used bandwidth has been validated in only four adult subjects, and we lack studies in children across different ages. Plus, she points out that fMRI and rs-MRI are actually identical in how the data are acquired during the scanning session, with one important difference that rs-fMRI do not involve a “task.” “We need grant opportunities and funding to do this leg-work before collecting data that may not be useable,” Dr. Denisova said.
Tissue structure. “Researchers typically assume that brain maturation and development occurs in a linear manner, but this is not correct,” Dr. Denisova said. First, typically developing newborns and infants show perceptual sensitivities to a variety higher-level stimuli, including discriminating between native vs. non-native language and detecting rhythm in music; newborns’ world is structured and cognition-ready. As a general point, we need to incorporate knowledge from the field of experimental cognitive developmental science into our brain imaging study designs. Second, certain brain substrates, including the cerebellum, already have microscopic myelin at birth; myelin sheathes neurons’ axons and facilitates the speed of neural communication. The main point is that different brain substrates do not mature in a similar way; some structures start off with a more advanced maturational profile and others exhibit protracted maturational trajectories during the first few years of life. This nuance suggests new insights for further work in atypical development in humans, including a test of Dr. Denisova’s optimality constraint theory of development. For instance, recent findings by the Denisova lab in the cerebellum showed that boys with ASD within a middle and late childhood age range (between 6 and 12 years) have different (flatter) structural properties of the right cerebellar cortex relative to typically developing boys.
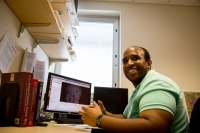
One goal of the current studies by Denisova’s team is to find out if atypically developing substrates contribute to atypical development, including ASD, in patients. “We are curious to find out if this atypical neuroanatomical pattern is present even earlier in development, in children at familial risk for autism,” Dr. Denisova said. “To this end, one advanced strategy to probe this question is what is called quantitative MRI (qMRI). This approach provides a measure of tissue relaxation values at each voxel and enables the study of tissue composition at the macro-level of analysis, compared to conventional (contrast-weighted) acquisitions.”
Dr. Denisova says her state-of-the-art guidelines are now out there for researchers to use and to consider as they plan MRI studies and analyses. The main take-home message for brain imaging studies with children is that data quality and data processing steps may impact conclusions and inferences. “In my view, now is an exciting time for new, transformative work in MRI worldwide, as we have many specific ways and opportunities to improve the methods in the field, an important consideration since high-field (7T) scanners are becoming more common in research settings,” she says. This is an important goal because at ultra high fields, not only the participant’s head movements, but also their body movements during data acquisition, disturb the heterogeneity of the magnetic field. Thus, several specific opportunities for new research include the development of optical prospective motion correction, as well as a call for studies that investigate the hemodynamic response function across different tasks in children. “We are also excited about the possibility of contributing towards development of new brain atlases for infants based on the concept of q-Maps (quantitative MRI maps) that would more precisely map function and structure in atypically developing children” said Dr. Denisova.
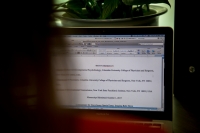
The article containing the new guidelines, “Neurobiology, not artifacts: Challenges and guidelines for imaging the high risk infant” is an Invited Contribution for the Special Issue of NeuroImage: Imaging Baby Brain, published online as Open Access before inclusion in the print issue: doi: 10.1016/j.neuroimage.2018.07.023.
A PDF of this article is also available.